Remember 2016
when Rayfield, Palmer and Martin-Silverstone of Bristol University created the following advertisement for a budding paleontologist/engineer student? “The main objective of this proposal is to investigate the effectiveness of the quadrupedal launch [of pterosaurs] and by comparing it with the bipedal launch of birds, test if it was one of the factors that enabled pterosaurs to become much larger than any bird, extant or extinct.”
The Bristol pterosaur workers
did not want to test a quad launch (Fig. 1) vs bipedal launch (Fig. 2) in pterosaurs. Rather this team assumed that pterosaurs took off on all fours based on the ability of tiny 1.2 oz vampire bats to leap and then unfold their wings, then flap, then fly.


Worse yet,
the Bristol team assumed giant azhdarchids could fly, ignoring the vestigial distal phalanges that made azhdarchids flightless before becoming giants. Engineer and inventor, Paul Macready, had to shorten the neck and lengthen the wings of his model Quetzalcoatlus to make it fly (Fig. 3).

I think the Bristol pterosaur team found the student they advertised for in 2016,
If so, he’s now a PhD: Benjamin W Griffin. This guess is based on the observation that this is the second time Griffin and his Bristol team have presented an abstract for pelvic range of motion during a hypothetical quad launch in pterosaurs.
From the Griffin et al. 2021 abstract:
“Pterosaurs were the first vertebrates to develop powered flight as a method of locomotion and subsequently attained sizes unseen in any other flying group.”
Only after becoming secondarily flightless, as described earlier here.
“Launch is the most power intensive aspect with regards to flight, requiring the generation of a launch impulse that will provide sufficient velocity and height to safely begin the flapping cycle. As flying animals increase in size, their capacity for muscular force generation does not increase at the same rate, making this launch impulse harder to generate.”
So far, that sounds sensible. That’s why birds combine a wing flap with a leg launch (Fig. 2). Unfortunately, the quad launch hypothesis separates these two methods for becoming airborne. Between the hind limb leap and the first flap of the pterosaur wing there is a long period of time in which there is no thrust and no lift (Fig. 1), only momentum.
“One hypothesis for how pterosaurs circumvented this issue is that they utilized a quadrupedal
launch, allowing them to incorporate the flight muscles in the launch cycle.”
The tiny bats that can leap into the air like this weigh only 1.2 ounces. Things are different when you are not so tiny (see video of fruit bat below). By contrast, birds the size of pterosaurs incorporate flight muscles in the launch cycle, combining the initial leap with energetic flapping to produce maximum thrust (Figs. 2, 4).
Griffin et al. continued:
“Ornithocheiraeans were a clade of Cretaceous pterosaurs including both Anhanguera and
Ornithocheirus. The largest members of this clade reached wingspans of 6 m. By using Range of Motion (ROM) mapping of the pectoral and pelvic girdles of a generalised 5 m wingspan ornithocheiraean model, we tested the ability of ornithocheiraeans to assume the poses required for quadrupedal launch.”
As the Griffin team did in 2019.
“Additionally, we were able to simulate the effects of soft tissues on the joint mobility of the girdles. This facilitates expansion of the ROM through cartilaginous offsets and restriction of the
ROM by constrictive soft tissues including ligaments and muscles. The ROM maps were then compared against published poses hypothesised to be used in pterosaur launch.”
Published poses? From paleoartists? Why not start from scratch and test quad poses against bipedal poses matched to tracks? See animated figure 4.
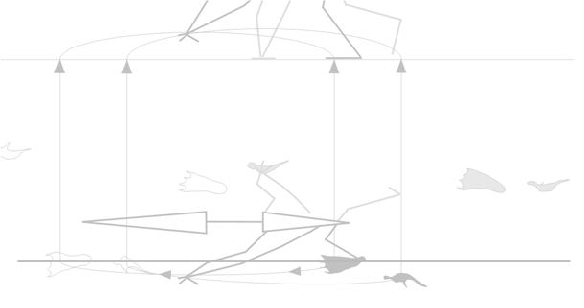
Wonder if the Griffin team ever got rid of the morphological cheats employed by Witton and Habib 2010 (Fig. 5), chronicled here in 2011. You might remember Habib’s idea was to plant the wing finger into the substrate, then roll over it to develop an elastic slingshot effect. The problem is: the wing finger never touches the substrate in any pterosaur track. To make pterosaurs do this Habib and Witton 2010 reduced the three free fingers and put them on top of the wing digit to get them out of the way (Fig. 5). That’s also called cheating. Strangely, no one else noticed as this hypothesis became adopted by museums and artists worldwide.
Griffin et al. continued:
“Over 197,000 potential poses were tested for the ball-and-socket joint of the pelvic girdle, and over 591,000 potential poses were tested for the more complex semihellar joint seen in the pectoral girdle.”
Methinks Griffin is ‘milking’ that 2016 offer made by co-authors Rayfield, Palmer and Martin-Silverstone and confessing to it! That is more poses than I would be interested in testing by about 196,900. BTW, the word ‘semihellar’ appears nowhere else in the English language according to a keyword search on Google.
“The ROM maps generated show that the ornithocheiraean model can assume the poses required for a quadrupedal launch.”
Apparently that is the resting (= pre-launch) pose (Fig. 6) for a quadrupedal ornithocheirid. Meanwhile, in the bipedal pose, the center of gravity remains over the extremely tiny (= vestigial) feet, which the animated version (Fig. 6 at left) illustrates much larger, again cheating morphology.
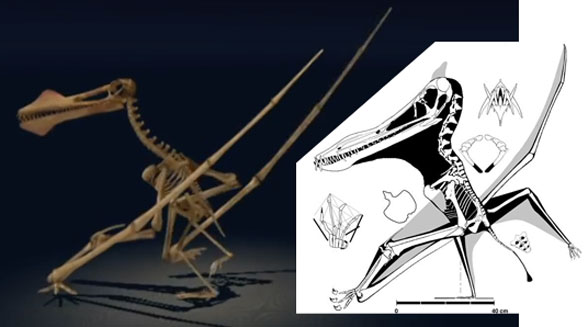
Griffin et al. continued:
“Additionally, the ROM maps indicate that the model is incapable of using a bipedal posture to generate a launch impulse, even with the largest cartilage offset.”
This will have to be demonstrated, not just said. Methinks they are cheating again in order to make the bipedal model invalid (or to keep their paychecks coming). Note how the model (Fig. 6 left) directs the femora nearly straight down, as if pterosaurs were archosaurs. The femora should be directed much more laterally, as in lepidosaurs, keeping the axis of the femoral head aligned with the axis of the acetabulum.
“This study demonstrates that medium sized pterosaurs could assume the postures required for quadrupedal launch. Next steps will estimate whether sufficient muscular leverage and
power could be generated through a quadrupedal launch cycle.”
Since this 2021 presentation repeats the one presented in 2019, the only cycle apparent here is the recycling of old myths and reconstructions. Scientists should want to falsify their hypotheses in order to get rid of those idea that don’t stand up to scrutiny. That’s when the harshest critics become the greatest allies, whether that feels good or not.
PS
Sharp-eyed readers will note that the university-level textbook “Vertebrate Paleontology” was written by another Bristol University professor, Michael Benton. In addition, David Unwin, who mistakenly imagined a single uropatagium between the hind limbs and lateral toes of Sordes from a displaced wing membrane, also taught at Bristol from 1991 to 1997. So if you wonder why myths persist in paleontology, look no further than this center of influence.
References
Griffin BW, Martin-Silverstone E, Demuth O, Pegas R, Palmer C and Rayfield E 2021. Pectoral and pelvic range of motion constraints on Ornithocheiraen quadrupedal launch. Journal of Vertebrate Paleontology abstracts.
Griffin BW, Demuth OE, Martin-Silverstone E and Rayfield EJ 2019. Simulated range of motion mapping of different hip postures during launch of a medium-sized ornithocheirid pterosaur. Journal of Vertebrate Paleontology abstracts.
Witton MP and Habib MB 2010. On the Size and Flight Diversity of Giant Pterosaurs, the Use of Birds as Pterosaur Analogues and Comments on Pterosaur Flightlessness. PLoS ONE 5(11): e13982. https://doi.org/10.1371/journal.pone.0013982